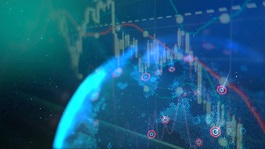
Publication
Global rules on foreign direct investment (FDI)
Cross-border acquisitions and investments increasingly trigger foreign direct investment (FDI) screening requirements.
Mondial | Publication | September 2016
This article focuses on the development of electrical energy storage alongside existing and future renewable energy projects. Current interest in this sector is primarily in lithium ion batteries, being driven by cost reductions, but a number of existing and developing technologies offer competition and the promise of lower costs.
As long ago as 2012, a number of manufacturers, developers and commentators endorsed the ambition of an additional 2,000 MW of energy storage by 2020 in the UK. According to one source, 362.8 MW of energy storage projects were announced worldwide in 2013-2014, with an almost equal distribution between North America, Asia Pacific, and Western Europe. Global installed energy storage for grid and ancillary services is expected to grow from 538 MW in 2014 to 21 GW in 2024. According to a 2014 report from Navigant Research, it is predicted that worldwide revenue from energy storage will increase from US$675 million in 2014 to US$15.6 billion in 2024.
Various Government and European Commission documents have identified the importance of energy storage for future energy systems. In the UK, the Department of Energy and Climate Change (DECC), before its integration with the Department for Business, Energy & Industrial Strategy, had suggested that there is scope for growth of storage up to 20 GW by 2050. In August 2012, the Low Carbon Innovation Coordination Group carried out a study suggesting that there is a potential range for UK storage deployment to 2050 between 7.2 GW and 59 GW, with 27 GW being the central estimate. A study by Imperial College suggests UK deployment of 25 GW of storage by 2050.
The increased capacity of generation from intermittent renewable energy sources poses technical issues for the UK electrical grid. Grid capacity is typically sized to match peak output from generators which are distributed around the UK grid. Energy storage has a role to play in managing output from generators and electrical grid control issues. Along with interconnection and demand side response, energy storage is a good means of smoothing peaks and troughs in generation. Storage can also help ease technical constraints such as frequency control, offsetting the need for grid reinforcement.
Conventional power generators typically have a single use; to create electricity. Energy storage has a variety of uses, which can be categorised as either “behind the meter” for distributed applications or “in front of the meter”, being more power grid orientated. In front of the meter storage could be located anywhere within the electrical transmission or distribution networks. Behind the meter storage can either be connected at a generator, to control output of the generator or to share the grid connection to provide other services, or connected at a consumer, to offer demand side response.
With this in mind, what are the key UK revenue streams for generators considering energy storage?
System frequency is determined and controlled by the real time balance between system demand and total generation. The frequency falls if demand is greater than generation. If generation is greater than demand, the frequency rises. National Grid has a licence obligation to control frequency. The limits specified in the 'Electricity Safety, Quality and Continuity Regulations 2002', are ±1% nominal system frequency (50.00Hz), save in abnormal or exceptional circumstances. This requires National Grid to ensure that sufficient generation and / or demand is available to manage frequency variations.1
FFR is designed to complement other types of Frequency Response and delivers firm availability. The service can be either dynamic – energy changes in line with system frequency – or static – energy change occurs at present frequency and remains at a set level.2 The tendered service of FFR is open to Balancing Mechanism Units (BMU), being the units of trade within the Balancing Mechanism used to balance supply and demand in each half hour trading period of every day, and to Non-BMU providers, thereby increasing the number of potential response providers and improving liquidity. FFR also creates a route to market for providers whose services may otherwise be inaccessible. The minimum power capacity to provide the FFR service is 10 MW. This may be from a single unit or aggregated from several smaller units. Units of less than 10 MW but planning to reach this threshold in the near future may be able to participate through a separate route.3
Suppliers can provide a Primary, Secondary or High Frequency Response if the frequency moves outside a deadband (±0.015 Hz) or past a pre-agreed level:
Tenderers may provide a combined Primary and Secondary static service. National Grid’s priority is for secondary response. National Grid procures the services through a competitive tender process, where tenderers can bid for low frequency events, high frequency events or both.5 National Grid will accept the most economical tenders. A successful tender then becomes contractually binding. The tenor of each bid varies, as tenderers are required to specify a “Tendered Service Period” when submitting their application.
Renewable energy generators with storage are able to participate in FFR, but must demonstrate a high level of availability of the service, typically requiring a permanent increase in grid import and export capacity for wind farms, or tendering only during hours of low generation for more predictable generators such as solar farms. National Grid nominates the hours of delivery to be tendered for 1 month in advance. The availability and nomination price is then set at a standard £/h rate for the duration.
Following a period of review in early 2016, National Grid has introduced a suite of complementary services to sit alongside the existing framework, which aim to increase flexibility and efficiency. Providers may now tender 1 month in advance, but nominate their MW a week ahead, on a settlement period basis. The associated price would therefore be on a £/MW/h basis. An associated pricing-related relief is the introduction of spread index tenders. his will replace the current fixed availability and nomination fee regime with spread indexed contracts, which will remove market risk by guaranteeing profitability at the nominated part load point. In relation to penalties, providers will now be able to (i) temporarily reduce their MW output without incurring a penalty; and (ii) avoid penalties backdated any further than 1 year from the event of default. In the interests of transparency, the company name and fuel type associated with each tenderer will be published to improve market efficiency. Finally, new, sub-sites will be able to be added more easily by providers to their existing frameworks without the need for any additional approvals or arduous documentation.6
EFR is a service that achieves 100% active power output at 1 second (or less) of registering a frequency deviation. This is in contrast with Primary and High Frequency FFR services which have response times of 10 seconds, and Secondary FFR which has a response time of 30 seconds. This is a new service that is being developed to improve management of the system frequency pre-fault, i.e. to maintain the system frequency closer to 50 Hz under normal operation.
In July 2016, National Grid procured 200 MW of EFR. Contracts were awarded subject to a 50 MW cap per pre-qualified party. Tenderers had to deliver an EFR service capable of operating at maximum charge or discharge for a continuous period of 30 minutes. The terms restricted committed EFR assets from concurrently providing a dual service, but EFR storage facilities were allowed to provide different services within a 24 hour period if not contracted to EFR at the specified times of the tender. The ability to provide dual service was also subject to prior approval by National Grid. Successful tenders underwent a two stage evaluation. Stage one required tenders to comply with the required application format. Stage two evaluated the tenders based on the total cost to National Grid rather than the unit price offered through the tender. Proposals were compared against the forecast cost of alternative action for the specified usage period of the provision of EFR.
The results of this process were published on 26 August 2016 with an average price of £9.44/MW per EFR/hour, as follows:
Bidder | Capacity procured (MW) | Price (£/MW of EFR/hour) |
---|---|---|
EDF Energy Renewables | 49 | £7.00 |
Vattenfall | 22 | £7.45 |
Low Carbon | 10 | £7.94 |
Low Carbon | 40 | £9.38 |
E.on UK | 10 | £11.09 |
Element Power | 25 | £11.49 |
RES | 35 | £11.93 |
Belectric | 10 | £11.97 |
Winning bids were at the lower end of expectations, suggesting that some bidders placed artificially low bids to gain first mover advantage or made more optimistic estimates of future revenue streams. National Grid offered 4 year contracts for EFR, which whilst less than the expected payback time of a storage system, provides greater certainty than for FFR.
The majority of the EFR capacity was won by storage providers, who benefited greatly from the objective of the tender, which despite being technology neutral, had at its heart decarbonisation of the traditional technical capacity fleet. The Renewable Energy Association’s head of policy and external affairs, James Court, highlighted the significance of this result, as the UK storage industry is now starting to deliver on its promise to become a world leader.
Renewable energy generators with storage are able to participate in EFR, but must demonstrate a high level of availability of the service, typically requiring a permanent increase in grid import and export capacity for wind farms, or tendering only during hours of low generation for more predictable generators such as solar farms.
The capacity market in the UK has been established to provide certain, regular payments to capacity providers, in return for which they must be available and producing electricity (or reducing demand) in times of system stress.
The capacity market could theoretically include electricity storage projects. The use of battery storage with a renewable energy source creates dispatchable generation allowing such systems which are of a suitable capacity and power output to participate in the capacity market. Energy storage must be of sufficient capacity to allow sustained power generation at the agreed level.
Successful tenderers are offered capacity agreements for different terms depending on whether plants are new or have been refurbished. To date the price set per kilowatt has been too low to justify capital investment in new energy storage projects. This has made it easier for existing nuclear and fossil fuel generators to offer ‘spare’ capacity into the auction at a much lower marginal cost. However, the capacity market may be considered as part of the business case for an energy storage project, as a follow-on revenue stream after contracts for more lucrative but short term ancillary services have expired.
Recent changes to the capacity market include lowering the minimum size of load reduction demand side response units to 500 kW, which should enable more storage projects to participate in the mechanism. Stronger penalties for not delivering capacity are also likely to help the mechanism’s attractiveness for storage projects. The mechanism continues to be criticised for supporting higher-carbon electricity generation.
Penalties are payable for failing to provide capacity. Providers with renewable sources and battery storage may consider not participating, as the potential open ended generating requirement may restrict them from delivering other ancillary services with higher revenue potential and means that tenderers must account for the cost of imported electricity to avoid the risk of financial penalties for not meeting their required output level.
STOR capacity is retained on stand-by,to be called on to generate within four hours of instruction. The need can arise from demand forecast errors, unexpected loss of thermal generation and variable wind generation. Availability payments are made during contracted windows and utilisation payments are made whenever reserve is delivered. STOR is open to transmission connected generation from large power station sites and small transmission or distribution connected generation and demand. National Grid procures STOR through a tender process. STOR projects are required to fulfil a number of criteria, including an ability to deliver at least 3 MW of reserve, an ability to react within 240 minutes of an instruction, an ability to deliver for a minimum of two hours, have a recovery period after provision of reserve of less than 20 hours, be able to provide reserve at least three times a week (though average utilisation will be much lower) and operational metering.
Renewable sources with energy storage can participate in this service, as storage permits dispatchable output for a period of time. It is possible to participate in this service individually or through an aggregator for smaller generators. While participation in this service should be considered, availability prices are significantly lower than for frequency response services.
Fast Reserve is a balancing service where the service provider delivers a contracted power output within agreed limits, when instructed to by National Grid. This is similar to the STOR service, but has a shorter timescale, with a much greater response rate of 25 MW/minute at a minimum. Fast Reserve provides rapid and reliable delivery of active power through an increased output from generation or a reduction in consumption from demand sources, following receipt of an electronic despatch instruction from National Grid. This service operates in quicker timeframes than STOR and requires a 50 MW minimum capacity. As such, it will only be suitable for larger projects or through an aggregator service.
To participate in this service a renewable source and battery storage system with sufficient large storage capacity and power output would be required. The power output from a battery energy storage system can be rapidly adjusted, far exceeding the ramp rate requirements. While participation in this service is possible using battery storage, the provider would likely be paid more for participating in services that more fully utilise the unique capabilities and very short response times of battery storage.
Generators who export during the three peak consumption periods may be eligible for Triad payments. National Grid selects three half hour periods of peak demand during the triad period. The triad period occurs between the months of November and February inclusive each year and each triad period is a minimum of ten days apart. The average of the maximum demand for customers over the three triad periods is used to calculate their Transmission Grid Use of System (TNUoS) charge for energy suppliers for the year, which they then pass onto their customers. Embedded generators within a Distribution Network Operator’s (DNO) grid can benefit by exporting energy during triad periods.
Renewable energy generators are well placed to install energy storage to improve the benefit from Triad payments as long as there is a pass through mechanism in the power purchase agreement pursuant to which generators sell electricity to electricity suppliers. Triad payments, along with other embedded benefits, are currently under review by Ofgem and are likely to change due to the increased proportion of embedded generation. It is unclear whether this benefit will continue to be available in its current form.
Super Red Credits are payments provided by DNOs to non-intermittent generators for exporting energy during peak demand times called super red periods. These payments are made as this generation allows the DNO to defer the reinforcement or upgrade of their grid. To receive these credits generators must be connected to their DNO’s Extra High Voltage (EHV) grid and be a non-intermittent generator. These credits are only applicable to generators in specific areas where their generation defers grid reinforcement. Participation in Super Red Credits payments is possible for renewable sources with battery storage. It allows the provider to produce non-intermittent generation and time shift energy generated by renewables at other times of day into the super red period to maximise its commercial benefit. Participation is only possible when the service provider is connected to a specific part of the distribution grid, which is reinforced by their generation.
To enter a non-intermittent power purchase agreement (PPA) the generator must be able to generate at an agreed power level for an agreed time period. A non-intermittent PPA also means renewable energy generators can receive a variable energy rate instead of a fixed one, making it beneficial to generate at peak times when a higher rate is available. A non-intermittent PPA can be considered as a long-term revenue source for a renewable source with energy storage, but the uplift in revenue is likely to be low compared to other end uses.
In order to maximise a project’s commercial position, it is possible to “stack” revenue sources. Energy storage is proposed to add flexibility to the electrical grid. Typically there is limited uplift in value from storing energy purely for dispatch at times of higher prices, and so energy storage systems must gain maximum revenue through the provision of flexibility.
The ability to stack revenue streams, and the optimal stacking solution, will be site and developer dependent, considering: the availability of the grid connection, the type, specification and applicability to each end use of the energy storage system, and the appetite for risk in depending upon future revenue streams which have relatively short contract durations. On occasions, storage projects may choose to be penalised for non-compliance with the conditions of one source of revenue in order to benefit from the rewards offered under another regime.
Some revenue streams can be combined by tendering services at different times, with priority given to higher value services. Other services will require additional power or storage capacity, which increases capital costs. Therefore the business case for each revenue stream can be tested against additional capital and operating costs applicable to that service. Furthermore, offering an additional service may reduce revenue available from another service due to mismatch in the technical or contractual requirements between services.
Navigating the complex array of criteria for each revenue stream is challenging. As the cost of energy storage drops, the need for revenue stacking to ensure a project’s economic viability is likely to reduce.
Electrical energy can be converted to many different forms of storage. These include: gravitational potential, compressed air, electrochemical in batteries, chemical, kinetic and magnetic. There is a range of technology maturity, application suitability, round trip efficiency and lifetime.
The dominant technology for battery energy storage is lithium-ion, due to its high energy and power density and reducing costs. These reducing costs are due to the development of lithium-ion technology for use in electric vehicles and personal electronic devices. Lithium-ion batteries made up 70 per cent of installed capacity for energy storage in 2015, with this likely to rise to over 90 per cent in 2016. Competing technologies are promising larger storage capacities at reducing cost.
The following table gives a high-level overview of some of the key technology types:
Technology | Description | Expected life (years) | Relative advantages | Relative disadvantages | Maturity | Round trip efficiency | Cycle limited | Response time |
---|---|---|---|---|---|---|---|---|
Lithium-ion |
Lithium-ion batteries are typical electrochemical cells comprised of a container, two electrodes, two current collectors, a porous separator and an electrolyte. Relatively established technology and used in electronics and electric vehicles |
5 - 15 |
|
|
Demo to Mature |
80 – 94% |
1,500 – 3,500 |
Milliseconds |
Lead acid batteries |
Lead acid batteries were the first type of rechargeable battery in commercial use and are reliable and inexpensive. Lead acid batteries are expected to have low applicability to commercial energy storage due to having relatively low energy density, slow recharge times and limited cycle life. However, some lead-acid battery technology combines standard lead-acid battery technology with ultra-capacitors; these technologies increase efficiency and lifetimes and improve partial state-of-charge operability. |
5 - 15 |
|
|
Mature |
75 – 80% |
300 -1,800 |
Milliseconds |
Sodium Sulphur |
Sodium sulphur batteries have energy densities much higher than lead-acid batteries as well as a longer cycle life and lifespan. This, combined with high efficiency and low self-discharge make Sodium-sulphur batteries suitable for longer term bulk energy storage, however they must be maintained at a temperature of 300° – 350°C |
5 - 15 |
|
|
Demo to Mature |
75-85% |
2,500 |
Milliseconds |
Flow batteries |
Flow batteries convert between chemical energy and electrical energy via a reversible electrochemical reaction between two liquid electrolyte solutions with dissolved metal ions. The electrolyte solutions are pumped through the electrochemical cell from storage tanks, hence the name ‘flow battery’. Flow batteries are defined by the electrolyte solution; vanadium redox and zinc-bromine are the most prevalent. |
15 - 20 |
|
|
Develop to Deployment |
70– 80% |
>1,000 - 104 |
Milliseconds |
Pumped hydro |
Primarily used for load-balancing, pumped storage stores energy by gravitational potential, using the difference in height between two reservoirs of water. During times of low demand water is pumped to a higher elevation for release at times of increased and peak demand. At release, water is directed through turbines to produce electricity. |
30-50 |
|
|
Mature |
65-75% |
2x104 – 5 x 104 |
Seconds to minutes |
Compressed air (CAES) |
Similar to pumped-storage hydro in terms of scale and utility, compressed air storage operates by compressing ambient air into underground caverns or in storage tanks. When electricity is required the pressurized air is released through an expansion turbine to generate electricity. Two methods of CAES can be utilised; the diabatic process utilising the exchange of heat and the adiabatic method which does not involve heat transfer processes. |
15 - 40 |
|
|
Demo to Mature |
65 - 75% |
104 – 3 x 104 |
Seconds to Minutes |
Flywheels |
Energy is stored by spinning a flywheel which is accelerated to a given rotational velocity (consuming energy), with energy extracted from the flywheel when needed by applying a load which decelerates the flywheel. Reducing frictional losses, by magnetically suspended flywheels in a vacuum, increases the efficiency of such devices. |
20+ |
|
|
Demo to Mature |
85 - 87% |
105 - 107 |
Instantaneous |
Gravitational potential |
Includes rail energy and gravity batteries. Advanced Rail Energy Storage has devised a system that uses energy to push a train of weighted cars uphill. To export power, cars are sent downhill, sending power back to the grid. Gravity batteries are formed from weights connected to a generator being lifted to store energy and released. |
20+ |
|
|
Develop to Demo |
75 - 85% |
- |
Seconds to Minutes |
Power-to-gas |
Excess electrical power can be used to produce hydrogen and oxygen via an electrolyser. Electrical energy can be retrieved by use of a fuel cell or direct combustion in a gas turbine or engine. Round trip efficiency of this cycle is low. |
5 - 15 |
|
|
Demo |
30 - 45% |
- |
Minutes |
Energy storage can be located either ‘behind the meter’ for distributed applications or ‘in front of the meter’, being more power grid orientated. In front of the meter storage could be located anywhere within the electrical transmission or distribution grids. Behind the meter storage can either be connected at a generator, to control output or sharing the grid connection to provide other services, or connected at a consumer, to offer demand side response.
A single energy storage system may provide multiple services. However, in practice, a selected technology and storage-to-power capacity ratio will be optimum for a limited number of end uses. The following table gives a high-level overview of some of the end uses for energy storage and which technologies are relevant:
Location | End use | Description | Selected relevant technologies | Selected conventional alternatives |
---|---|---|---|---|
In front of meter |
PV / Wind integration |
Energy storage designed to reduce potential integration challenges. Longer term storage has potential for arbitrage. |
Lithium-Ion, Flow Battery, Pumped Hydro, CAES |
Transmission / distribution upgrade |
Ancillary services frequency regulation |
Import and export of power to provide services, including:
|
Lithium-Ion, Flywheel |
Gas turbine |
|
Transmission system |
Large-scale storage to improve transmission grid performance and assist in the integration of large-scale renewable generation |
Lithium-Ion, Pumped Hydro, Flow Battery, CAES |
Transmission line upgrade |
|
Distribution system |
Energy storage at substations to provide peaking capacity and mitigate stability issues |
Lithium-Ion, Flow Battery |
Distribution system upgrade |
|
Peaking plant replacement |
Large-scale storage designed to replace peaking facilities |
Lithium-Ion, Flow Battery, Pumped Hydro, CAES |
Gas turbines |
|
Behind meter |
PV / Wind integration |
To reduce potential integration challenges, reduce grid connection capacity requirement, make generator non-intermittent, provide ancillary services |
Lithium-Ion, Flow Battery |
Distribution system upgrade Reduce PV/wind capacity Curtailment / Balancing Mechanism |
Micro / Island Grid |
To enhance the stability of a microgrid system with specific local goals, such as reliability, diversification of energy sources and cost reduction |
Lithium-Ion, Lead-Acid, Flow Battery |
Diesel reciprocating engine Gas turbine Load profile alteration |
|
Commercial and industrial |
Energy storage system primarily designed to provide demand-side reduction for commercial or industrial applications. |
Lithium-Ion, Lead-Acid, Flow Battery |
Diesel reciprocating engine Utility service upgrade Load profile alteration |
Energy storage facilities will most likely be consented by way of a planning permission pursuant to the Town and Country Planning Act 1990 (‘TCPA 1990’) or by way of a development consent order (‘DCO’) pursuant to the Planning Act 2008 regime, depending on the scale of the facility.
The Planning Act 2008 introduced a new planning system for nationally significant infrastructure projects requiring that a DCO is obtained for electricity generating stations with a capacity of more than 50 MW. DCOs are granted on application to the Secretary of State.
By way of example, Snowdownia Pumped Hydro Limited has made a DCO application to construct a pumped storage facility with an output capacity of 99.9 MW in Glyn Rhonwy, Wales, which is currently under examination. By contrast, the local grid operator UK Power Grids is trialling a 6 MW battery storage facility (the Smarter Grid Storage facility) in Leighton Buzzard for which it obtained planning permission under the TCPA 1990 in 2013.
It is possible that the development of certain energy storage facilities (or development associated with such facilities) may benefit from permitted development rights if it is carried out by a DNO as statutory undertaker on ‘operational land’, as per the Town and Country Planning (General Permitted Development) (England) Order 2015. ‘Operational land’ in relation to statutory undertakers is land which is used for the purpose of carrying on the undertaking and in which an interest is held for that purpose. For instance, the installation of underground electric cables and the extension or alteration of buildings is permissible, subject to certain restrictions. This assumes that the addition of the energy storage facility on ‘operational land’ does not constitute a material change of use from the pre-existing operations and can be classed as ancillary development.
A key factor to consider will be sourcing appropriate locations for energy storage facilities. The Government’s Overarching National Policy Statement for Energy (EN-1) published in July 2011 (in accordance with which applications for DCOs must be determined) noted, in respect of pumped storage, that ‘there is limited further potential in the UK due to a lack of appropriate locations’. However, UK Power Grid notes that ‘it is unlikely that the scale of most distribution-connected energy storage would fall into the category of nationally significant infrastructure’ and, as such, there may be more scope for smaller-scale energy storage facilities brought forward pursuant to the TCPA 1990 regime. The Smarter Grid Storage Facility in Leighton Buzzard was constructed adjacent to an existing UK Power Grids primary substation site and DNOs may consider options at or nearby existing ‘operational land’ in the first instance.
Energy infrastructure is attracting substantial interest not only from traditional banks, but also from institutional investors such as insurance and pension funds. A key consideration to be addressed at an early stage will be how many of these investors will be prepared to take new technology risk and revenue stream uncertainty on financing energy storage. It will be important to structure energy storage projects so as to maximise the flexibility for future financing options.
The bankability of an energy storage project will depend to a high degree upon the main revenue source available to the project, its duration and the creditworthiness of the payment counterparty. In terms of ancillary services provided to National Grid, contract duration is insufficient to entirely repay construction loans for a new build energy storage asset and so developers may have to rely upon multiple future revenue streams. Another consideration is the project’s exposure to penalties for non-delivery of power. Monthly and/or annual liability caps in incentive regimes can limit downside risk and facilitate financing.
The interface between different parts of the revenue stack will need to be understood and the project’s revenues structured to respect market rules. Significant technical advice is likely to be required. The structure of storage contract terms will impact the risk profile of energy storage projects. For example, a 12 month contract can be structured as 12 one month contracts rather than one 12 month contract. This is relevant as owners will be concerned that one significant performance issue in a month does not incur the risk of losing the entire contract.
A key issue over the next few years is likely to be the extent to which storage applications can be readily combined with renewable energy generators (existing or planned). In the UK, almost all existing projects are likely to be supported by renewable energy incentive regimes such as the Renewables Obligation (RO), Feed-in Tariffs (FITs) or Contract for Differences (CfDs). This gives rise to a number of considerations:
It will need to be determined whether any storage element will be part of the original project, or will be set up, for example, under its own special purpose company, with completely separate contractual arrangements, and with its own access to revenue sources. Issues on which it may be difficult to achieve complete separation include access to land rights, grid connection arrangements and impacts on metering (which can impact support regimes).
Existing lenders will be unlikely to consent to any change to the project and/or its contractual arrangements that could potentially have a negative impact on the project or its revenues. They will need assurances that there will be no impact on important aspects of the project such as its accreditation under a support regime, cashflows, or grid connection agreements.
Storage facilities will also need to secure the relevant land rights.
Whilst many current project leases include wording that is broad enough to allow the installation of storage facilities without requiring landlord consent the layout of each project will dictate whether new land rights will be required where storage facilities are being located alongside existing renewable energy projects.
Cabling from a generating asset to a storage asset located on the current demise may not require landlord consent but it is likely that the route to grid cabling will do so (either from the landlord or any third party landowners). The position will certainly be simpler for projects where the point of connection is located on the landlord’s premises as the lease will likely already allow for the installation of cabling on the premises and the landlord’s retained land (subject always to planning and any other third party/title requirements).
Where a project envisages the use of a third party storage facility provider then an underlease may be needed – typically that would require landlord consent. As always and as above, an additional consideration is whether the installation of a storage assets alongside existing renewable energy projects will require planning consent.
It will not always be technically possible for a project’s export connection to be converted to an import connection. Where it is the intention that existing grid capacity is to be used more efficiently with the addition of a storage facility, it is likely that a grid cooperation agreement will need to be put in place between the generation and storage assets. This could cover issues such as land rights, access and forced de-energisations.
A related but separate issue is the interaction of storage with renewables incentive regimes. Neither the RO, FITs nor CfDs expressly prohibit renewables energy projects benefiting from support from engaging in storage. However, DECC has recently consulted on how low carbon projects that will be supported under the CfD regime might integrate storage. They identify that if generators wish to import electricity from the grid to store electricity and subsequently sell when prices are high they will need to ensure that this imported electricity is excluded from the balancing mechanism unit metered volume used to calculate CfD payments. DECC has proposed that that storage should be in a different BMU to the generation assets. They have also proposed a definition of “storage facility”. Similar concerns in respect of metering electricity would apply in the context of RO and FIT-supported projects.
Where storage projects will be co-located, alongside existing projects, this is likely to impact metering arrangements. Under the RO, changes to metering set-up can be agreed with Ofgem and a project’s accreditation updated. However, this process gives rise to delays in Renewables Obligation Certificates issuance, and the risk that an updated accreditation is issued pursuant to conditions that are unworkable.
Given the potential variety of options for energy storage, each project is likely to have a different optimum configuration, power, capacity and potential revenue streams, dependent upon factors such as whether or not it is co-located with a renewable energy generator or a greenfield/standalone project, existing/available grid connection capacity (both export and import), the project’s location and the risk appetite of its investors.
Despite the lack of clear regulatory policy to encourage accelerated market penetration of energy storage technologies in the UK, cost reductions through higher efficiencies and a reduction in the use of expensive materials will lead to further substantial growth in the near- and long- term. The current price of grid-scale batteries makes them commercially well suited to perform short duration and high power functions (as evidenced in the ancillary services market). The potential of further substantive cost reduction is expected to facilitate bulk energy storage in the future. When combined with renewable energy generation this will reduce the need for non-intermittent fossil fuel power systems and base-load power systems.
This article was written in conjunction with Chris Parcell (Director of Feasibility and Development) at SgurrEnergy
Source: National Grid ‘Firm Frequency Response Guide’, May 2015
Source: National Grid, ‘Firm Frequency Response: Frequently Asked Questions’, May 2015
Publication
Cross-border acquisitions and investments increasingly trigger foreign direct investment (FDI) screening requirements.
Publication
Artificial intelligence (AI) raises many intellectual property (IP) issues.
Publication
We are delighted to announce that Al Hounsell, Director of Strategic Innovation & Legal Design based in our Toronto office, has been named 'Innovative Leader of the Year' at the International Legal Technology Association (ILTA) Awards.
Subscribe and stay up to date with the latest legal news, information and events . . .
© Norton Rose Fulbright LLP 2023